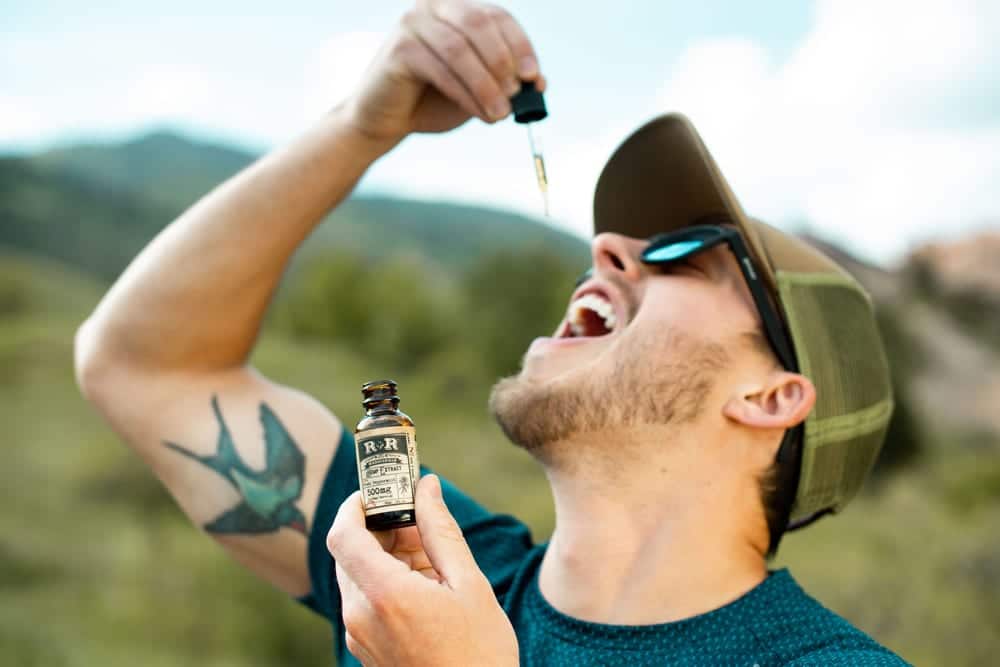
CBD Drug Interactions
CBD Drug Interactions
Every day people are looking to see if CBD can be used with their existing medication, or in fact replace it. This sections explores research published about the use of CBD interactions with popular medication. The information below should not be interpreted as an instruction or medical advice to displace the advice provided by your physician or other medical professionals. We recommend consulting with your doctor or other qualified health professionals for the preparation of a treatment plan for any diseases or ailments. Do not solely rely on the information provided by CBD Clinicals.
CBD and Zoloft (Sertraline)
Can CBD be taken with Zoloft? According to Mayo Clinic, antidepressants are a popular treatment choice for depression. Although antidepressants may not cure depression, they can reduce the symptoms(4). Many
Read moreCBD and Xanax
Can CBD be taken with Xanax? Alprazolam (Xanax) is a benzodiazepine. Benzodiazepines are categorized under a group of medicines called central nervous system (CNS) depressants, which are medicines that calm
Read moreCBD and Metronidazole
Metronidazole is an antibiotic that belongs to the nitroimidazole class of antibiotics. Can CBD be taken with Metronidazole?
Read moreCBD and Opioids – CBD Clinicals
The United States is beset with an opioid epidemic that, so far, has claimed more than 300,000 lives. The analgesic effect of opioids declines and tolerance builds up over time.
Read moreCBD and Caffeine
The caffeine content of beverages varies widely, and coffee is the primary source of dietary caffeine consumption. The International Coffee Organization says coffee lovers consume approximately 1.6 billion cups of
Read moreCBD Rosin
Hemp pressing provides CBD users an excellent opportunity to experience making their CBD rosin themselves. If one is not into the DIY rosin experience, CBD rosin is available on the
Read moreCBD and Warfarin
Can CBD Be Taken with Warfarin? It is not recommended to take CBD with warfarin. Warfarin (brand names: Coumadin, Jantoven) is an oral anticoagulant or blood thinner that is primarily
Read moreCBD and Nicotine
Nicotine is a synthetic chemical that contains nitrogen, which is produced by numerous types of plants, including the tobacco plant. When tobacco is smoked, nicotine activates the release of dopamine,
Read moreCBD Toxicity
Toxins are organic poisons produced by animals, plants, or bacteria and can cause certain diseases. Research shows that toxins have become a primary cause of chronic disease. Toxins include not
Read moreCBD and Folic Acid
What is Folic Acid? Vitamin B9, also referred to as folic acid, helps the body produce and maintain new cells. The synthetic form of folate, folic acid is used in
Read moreCBD and Aspirin
Can CBD Be Taken with Aspirin? The cytochrome P450 enzyme system, which is found within the liver, is responsible for metabolizing (breaking down) potentially toxic compounds, including over 60% percent
Read moreCBD and Metformin
Can CBD Be Taken with Metformin? Metformin is a U.S. Food and Drug Administration (FDA) approved medicine commonly used to treat type 2 diabetes. Doctors often prescribe this drug on
Read moreCBD and Cocaine
Does CBD Interact with Cocaine? What is Cocaine? Cocaine is a strongly-addictive stimulant drug that can have a detrimental effect on the mental health of the user. It is brewed
Read moreCBD Oil and Benadryl
Does CBD interact with Benadryl and other antihistamines? Benadryl is the brand name for a substance known as diphenhydramine HCL. Diphenhydramine belongs to a class of medications called antihistamines.
Read moreCBD and Alcohol
A study in the journal Psychopharmacology stated that taking CBD with alcohol resulted in significant impairments of the test subjects’ psychomotor and motor performances
Read moreCBD and Tramadol
Tramadol has been used as a pain reliever for years. However, because of its potential for abuse, it may not be for everyone. CBD has been shown as a safer
Read moreCBD and Ivermectin
Ivermectin has been used to treat and control parasitic infections caused mainly by parasitic worms known as nematodes (except tapeworms) for more than 35 years. CBD, on the other hand,
Read moreCBD Oil and Antibiotics
Antibiotics are used to prevent or treat specific types of bacterial infections. In some cases, they are prescribed for an infection that keeps coming back or causes an increased risk
Read moreCBD Oil and Magnesium
Does CBD Interact With Magnesium? Magnesium is a mineral naturally present in a variety of foods, available as a supplement, and an ingredient in antacids and laxatives
Read moreCBD Oil and Prednisone
Does CBD interact with prednisone, and if so, are there risks that should cause concern? Prednisone is a prescription drug used to provide relief for inflamed areas of the body.
Read moreCBD and Zofran (Ondansetron)
Can one take CBD with Zofran, and are there any interactions that should cause some concerns? Find out how CBD can help with nausea, vomiting, and other health issues. Is
Read moreJoin our
newsletter and
never miss a beat.
We do all the research,
so you don’t have to.